INSTANT, International Nusantara STratification ANd
Transport program
1. Introduction
The Indonesian seas provide a low-latitude pathway for the
transfer of warm, low salinity Pacific waters into the Indian Ocean.
This Indonesian Throughflow (ITF) plays an integral
part in the global thermohaline circulation and
climate phenomenon (see Sprintall et al., 2001 and
Gordon, 2001 for recent overviews; Fig. 1), and the heat and freshwater carried
by the ITF impacts the basin budgets of both the Pacific and the Indian
Oceans (Bryden
and Imawaki, 2001; Wijffels,
2001; Wajsowicz and Schneider, 2001). Within the internal
Indonesian Seas,
observations and models indicate that the primary ITF source is North Pacific thermocline water flowing through Makassar Strait (sill depth 650 m). Additional
ITF contributions of lower thermocline water and deep
water masses of direct South Pacific origin are derived through the eastern
routes, via the Maluku and Halmahera Seas,
with dense water overflow at the Lifamatola Passage
(sill depth 1940 m). The ITF exits into the eastern Indian Ocean
through the major passages along the Lesser Sunda Island chain: Ombai Strait
(sill depth 3250 m), Lombok
Strait (300 m), and Timor Passage
(1890 m). The complex geography of the region, with multiple narrow
constrictions connecting a series of large, deep basins, leads to a circuitous
ITF pathway within the Indonesia
seas. Enroute the Pacific inflow waters are modified
before export to the Indian Ocean due to mixing,
upwelling and air-sea fluxes.
In recent years a number of monitoring programs have measured
aspects of the ITF from its Pacific source, through the internal seas, to the
exit passages. The programs range from individual year-long mooring deployments
in Makassar, Timor and Ombai
Straits, a three-year shallow pressure gauge array (SPGA) in the exit passages,
to decade long XBT transects within the Indonesian region and five full-depth
hydrographic CTD/ADCP sections between Australia and Indonesia. A serious
shortcoming in the recent measurement programs within the Indonesian region is
the lack of temporal coherence: the data cover different time periods and
depths in the different passages of the complex pathways toward the Indian
Ocean. This has lead to ambiguity of the mean and variable nature
of the ITF, and the transformation of the thermohaline
and transport profiles within the interior seas. For this reason, and in
keeping with the recommendations stemming from the full community deliberation
(OCEANOBS October 1999 meeting in St. Raphael, see Imawaki
et al., 1999; Workshop on Sustained Observations for Climate of the Indian
Ocean, Perth, November 2000, see CLIVAR Exchange, 3:4, December 2000 and
www.marine.csiro.au/conf/socio/socio.html), an international co-operative
effort is planned to deploy multi-year moorings for direct Throughflow
velocity, salinity and temperature measurements simultaneously in Makassar Strait, Lifamatola
Passage, Lombok Strait, Ombai
Strait and Timor Passage (Table 1). The program called INSTANT (International Nusantara STratification ANd Transport) involves 5 nations:
Australia, France,
Indonesia, Netherlands,
and the USA.
This collaborative proposal by Lamont-Doherty Earth Observatory (LDEO) and
Scripps Institute of Oceanography (SIO) represents the U.S.
effort of the international INSTANT experiment listed in Table 1. Letters of
Intent from the other countries are included as appendices.
Figure
1. Schematic
of Indonesian Throughflow pathways (Gordon, 2001). The solid arrows represent North Pacific thermocline water; the dashed arrows represent South
Pacific lower thermocline water. Transports in Sv
(106m3s-1) are given in red. The 10.5 Sv in
italics is the sum of the flows through the Lesser Sunda
passages. ME is
the Mindanao Eddy; HE is the Halmahera Eddy. Superscript refers to reference source: 1: Makassar Strait
transport in 1997 (Gordon et al., 1999); 2: Lombok
Strait (Murray and Arief, 1988; Murray et al., 1989)
from January 1985 to January 1986; 3: Timor Passage (between Timor and
Australia) measured in March 1992 to April 1993 (Molcard
et al., 1996); 4: Timor Passage, October 1987 and March 1988 (Cresswell et al., 1993); 5: Ombai
Strait (north of Timor, between Timor and Alor
Island) from December 1995 to December 1996 (Molcard
et al., 2001); 6: between Java and Australia from 1983 to 1989 XBT data (Meyers
et al., 1995; Meyers, 1996); 7: Upper 470 m of the South Equatorial Current in
the eastern Indian Ocean in October 1987 (Quadfasel
et al., 1996); 8: Average ITF within the South Equatorial Current defined by 5
WOCE WHP sections (Gordon et al., 1997).
The hollow arrow represents overflow of dense Pacific water across the Lifamatola Passage into the deep Banda Sea,
which may amount to about 1 Sv (van Aken et al., 1988).
Inserts A-D show positions of INSTANT moorings.
Insert A: 2 Makassar Strait Inflow moorings (U.S.,
red diamond) within Labani Channel. Insert C: Netherlands mooring within the main channel of Lifamatola Passage (yellow triangle). Insert B, D: Sunda moorings in Ombai Strait,
Lombok Strait,
and Timor Passage (U.S.,
red diamonds; France,
purple square; Australia,
green circles). The positions of the
shallow pressure gauge array (U.S.,
green X). The 100, 500, and 1000 m
isobaths are shown in the inserts.
The collective merit for an internationally coherent program
has become strongly apparent given the complexity and scope of the disparate
nature of the present observations. INSTANT is designed to provide a time
series of ITF transport and property fluxes, and their variability from intraseasonal to annual time scales, along the ITF pathway
from the intake of Pacific water at Makassar
Strait and Lifamatola Passage, to the
Lesser Sunda exit channels into the Indian
Ocean (Fig. 1). The international group of PIs
and their respective responsibilities as part of the INSTANT program are
listed in Table 1. All the PIs bring their own valuable scientific expertise to
the project, and all have established good scientific track records of working
in the Indonesian region on Throughflow problems,
both individually and collaboratively as a team. Many of the supporting
measurements of the INSTANT program, such as the multi-year time series from
the Indonesian tide gauge and meteorological network, and the Australian XBT
program, already exist and are ongoing. The combined data set from the
international INSTANT mooring experiment and the supporting observational network, will leverage our individual measurements to
understand the broad spectrum of variability in the ITF, and the role that
regional oceanography plays in establishing the transfer function between the
Pacific inflow and the outflow into the Indian Ocean.
Table 1. Components of INSTANT
|
ITF Mooring Components:
|
Makassar Strait
|
USA
|
Gordon, Ffield, Susanto
|
Lombok Strait
|
USA
|
Sprintall
|
Ombai Strait
|
USA
|
Sprintall
|
Timor
Passage
|
Australia
|
Wijffels
|
Timor
Passage
|
France
|
Molcard, Fieux
|
Lifamatola Passage
|
Netherlands
|
van Aken
|
Supporting/Proxy Measurements:
|
CTD and
Underway ADCP
|
Indonesia
|
BPPT
|
Lesser Sunda Pressure Gauges
|
USA
|
Sprintall
|
XBT Network
|
Australia
|
Wijffels, Meyers
|
Tide Gauges
|
Indonesia
|
Bakosurtanal (survey and
mapping)
|
Meteorological Network
|
Indonesia
|
Badan Meteorologi
dan Geofisika
|
ARGO Floats
|
Australia
|
Wijffels
|
Ship Facilities:
|
|
|
Baruna Jaya
|
Indonesia
|
BPPT
|
The two Makassar
moorings build upon the results of the December 1996 to July 1998 Arlindo Makassar moorings that
were deployed in the Labani Channel, a 45 km wide,
2000 meter deep, constriction near 3°S (Fig. 1).
Frictional considerations and local wind processes therefore lead to very
little throughflow contribution over the very shallow
coastal flats to the west of the moorings (Fig. 1). The INSTANT moorings in Makassar Strait will be deployed at the same
sites as the Arlindo moorings, within the deep Labani channel. The Lifamatola Passage mooring will resolve the lower thermocline and deeper water inflow into the Banda
Sea. The Sunda observations in Lombok Strait,
Ombai Strait,
and Timor Passage, will capture the ITF as it exits from the interior seas into
the Indian Ocean. Most moorings will be instrumented
with upward-looking ADCPs to measure velocity of the
surface layer. The Makassar
and Lifamatola moorings will also have downward
looking ADCPs to measure the deep, dense water
overflows. The moorings will have a relatively dense suite of discrete
velocity, temperature and salinity measurements designed to resolve the net ITF
property transports.
The initial deployment of all moorings will be in August
2003, with re-deployment in February 2005, and recovery in July 2006. The
resulting simultaneous, multi-year direct measurements in both the inflow and
outflow ITF passages will allow us, for the first time, to unambiguously
determine the magnitude of inter-ocean transport, its properties, and their
evolution between the Pacific and the Indian
Oceans. Finally, an important
outcome of the program will be to recommend an efficient set of ongoing
observations that may offer a proxy for an efficient long-term monitoring
strategy of the ITF transport and property fluxes.
2. Scientific Objectives of INSTANT
2.1 The
Global Perspective: To determine the full depth velocity and property structure
of the Throughflow and its associated heat and
freshwater flux.
Large-scale observation based studies (including inverse
solutions) reveal significant Pacific export of mass, heat and freshwater into
the Indian Ocean (Piola and
Gordon, 1984, 1986; Toole and Raymer, 1985; Wijffels et al., 1992; Toole and Warren, 1993; MacDonald,
1993; MacDonald and Wunsch, 1996; Ganachaud
et al., 2000; Ganachaud and Wunsch,
2000). Uncertainty in the size of such a warm, fresh Throughflow
is the dominant source of error in analyses of the basin-wide budgets of heat
and freshwater for the Pacific (Wijffels et al.,
2001) and Indian Oceans
(Robbins and Toole, 1997). Models reveal dependence of Pacific and Indian Ocean
SST and upper layer heat storage on the throughflow (Hirst and Godfrey, 1993; Verschell
et al., 1995; Murtugudde et al., 1998). The Indian
and Pacific Oceans
would be very different if the ITF were zero (MacDonald, 1993; Schneider, 1998;
Maes ,
1998; Wajsowicz and Schneider, 2001). Oceanic heat
and freshwater fluxes into the Indian Ocean - at the
expense of the Pacific - affect atmosphere-ocean coupling with potential
impacts on the ENSO and monsoon phenomena (Webster et al. 1998). SST anomalies
which lead to a dipole mode of variability in the tropical Indian
Ocean first appear in the vicinity of Lombok Strait
in Indonesia (Saji et al., 1999).
The cold SST anomaly shifts westward along the Java and Sumatra Indian
Ocean coast and varies in strength with the phase of ENSO (Susanto
et al., 2001). The ITF source water (North Pacific vs. South Pacific) depends
upon land geometry and the tropical Pacific wind fields (Nof,
1996; Wajsowicz, 1999; Morey et al., 1999; Cane and
Molar, 2001). Observations show that the ITF is composed mostly of North
Pacific water flowing through Makassar
Strait (Fine, 1985; Ffield and Gordon,
1992; Gordon, 1995; Gordon and Fine, 1996), consistent with theory (Wajsowicz, 1996).
Makassar
Strait Moorings: The INSTANT moorings in Makassar Strait will be deployed at the same
coordinates as the 1996-1998 Arlindo moorings. The Arlindo moorings provided current and temperature time
series at various depths (Fig. 2) at two moorings within Labani
Channel of Makassar Strait
between December 1996 and June 1998 (Gordon et al., 1998; Gordon and Susanto, 1999; Gordon et al., 1999; Ffield
et al., 2000; Susanto et al., 2000; Vranes et al. 2002). The 1997 average southward transport
in Makassar Strait is 9.3±2.5 Sv.
The highest southward flow occurred at mid to lower thermocline depths (Gordon and Susanto,
1999; Gordon et al., 1999). The subsurface maximum occurs during times of large
transport, from April to September 1997 and again in April 1998 to the end of
the record in June 1998. An upward looking ADCP at 150 m on the Arlindo Makassar Strait moorings, provided a record of surface layer flow from 1 December 1996 to 1 March 1997. This time series shows
increasing southward speeds with increasing depth, while the surface flow
varied from near zero to northward. Attesting to the complex velocity profile, Waworuntu et al. (2001) - using TOPEX/POSEIDON altimeter
data, and data from 2 pressure sensor/inverted echo sounders deployed in Makassar Strait as part of the Arlindo
1996-1998 array - find that a three layer model of the transport profile is
required to explain the Makassar dynamics, with the
middle layer (roughly 200-500 m) providing a significant contribution to the
total transport. The subsurface velocity maximum through Makassar Strait results in a low transport-weighted
temperature of 11.5°C,
with an average salinity of 34.45 (Vranes et al.,
2002). This is cooler and saltier than the ITF characteristics envisioned by Piola and Gordon (1984; ITF salinity of 33.6), and Toole
and Warren (1993; ITF temperature of 24°C). In the southeast Indian
Ocean, the transport-weighted temperature over the top 650 db
across the IX1 XBT line is ~17.1°C, from the 15-year time series (Wijffels
and Meyers, pers. comm.). Warming between the inflow
at Makassar and the export
into the Indian Ocean is likely a consequence of the
impact of mixing, air-sea fluxes and upwelling within the internal Indonesian
seas.
While some of the Makassar
throughflow exits the Indonesian
Sea within Lombok
Channel (Murray and Arief, 1988), most turns eastward
within the Flores Sea
to enter the Banda Sea before entering the Indian
Ocean via the deeper Ombai
Strait and Timor Passage (Gordon
and Fine, 1996). The Banda Sea receives small amounts of
South Pacific lower thermocline and intermediate
layers by way of the Maluku and Halmahera Seas
(Ilahude and Gordon, 1996; Gordon and Fine, 1996; Ffield and Gordon, 1996; Hautala
et al., 1996; Kashino et al., 1996; Cresswell and Luick, 2001; Luick and Cresswell, 2001). It
may be that some Makassar
transport flows northward east of Sulawesi
to return to the Pacific Ocean, but it is unlikely that
this is a significant amount: water mass analysis of the Arlindo
data (Gordon and Fine, 1996) in that region does not indicate northward flow
within the thermocline. Northward transport may occur
in the surface layer, which may be composed in part of upwelled
Makassar water in the Banda
Sea. Annual average upwelling within the Banda Sea
is about 0.75 Sv (Gordon and Susanto,
2001). If half of the upwelling flows
northward, less than 5% of the Makassar
transport may return to the Pacific Ocean in the surface
layer, not a significant amount. At depth, the Banda Sea
is flooded with overflow of South Pacific waters across the 1940 m deep Lifamatola Passage (Jonker et
al., 1986; van Aken et al., 1988). The ITF
contribution through the Lifamatola Passage will be
monitored by a full-depth mooring, contributed by the Netherlands.
Recent ADCP cross-sections of the upper 250 m in Lombok Strait
(Fig. 4) distinguish a more variable upper layer reaching to 100 m (with
northward flow in December 1995), from a deeper layer that displayed constant
southward velocities of 20-60 cm/s (Hautala et al.,
2001). In all three survey years, the strongest outflow is found near Bali,
on the western side of the strait. Time series of temperature and salinity,
co-located at the pressure gauge sites in Lombok Strait,
consistently find the Bali side to be cooler and saltier
compared to Lombok. The
distinct behavior of the upper/lower layers, and the quasi-permanent western
intensification was also observed at the northern moorings during the southeast
monsoon in the Lombok Strait Experiment (Murray and Arief, 1988; Arief, 1992). In
fact the oft-cited average transport through Lombok Strait
of 1.7 Sv (Murray and Arief,
1988) was determined as an average of current meter data from either side of
the channel for 1985, although the western (Bali)
mooring was only instrumented after June 1985. Their reported transport curve
may therefore tend to underestimate the amplitude of the outflow pulse
occurring near the end of the southeast monsoon, and consequently the annual
average as well.
Ombai
Strait lies between Alor and Timor Islands,
and connects the Banda Sea with the Savu Sea
(Fig. 1). The strait is 30 km wide, and a recent bathymetric survey by Molcard et al. (2001) suggests a sill depth of 3250 m. From
a single mooring in Ombai
Strait, Molcard
et al. (2001) suggest a transport range between 4.3 Sv
and 5.8 Sv, depending on the assumed cross-strait
shear, with 0.9-1.2 Sv average transport in the upper
100 m. Although the deeper currents between 500-1220 m are weak, they still
contribute to the transport and appear uncorrelated to surface variability, and
thus must be independently measured.
The flow measured in Ombai Strait
was directed westwards towards the Savu Sea
(and Indian Ocean) during the entire mooring deployment,
except at the beginning of the record in December 1995, when flow reversed in
the upper 120 m towards the Banda Sea (Molcard et al., 2001). December 1995 coincides with the
northward surface flow observed in Lombok Strait
(Fig. 4), and the eastward surface flow across Sumba Strait
at the western edge of the Savu
Sea (Hautala
et al., 2001). The reversed surface flow during December 1995 is most likely
due to the seasonal eastward incursion of the South Java Current associated
with the passage of the coastal Kelvin wave passing into the Savu Sea
and on through Ombai
Strait. Indeed, cross-correlations
of the pressure gauge time series during December 1995, show the eastward
propagation from Bali to Sumbawa (northern Sumba Strait) to the Ombai Strait
gauges, with a speed of roughly 2.5 m/s, commensurate with linear wave theory (Chong et al., 2000). ADCP surveys across Ombai Strait
in both March 1997 and March 1998 show the most persistent westward Throughflow in these passages is mainly confined to the
southern two-thirds of the straits (Fig. 4). Thus at least two INSTANT moorings
are required in Ombai
Strait in order to capture the
cross-passage variability.
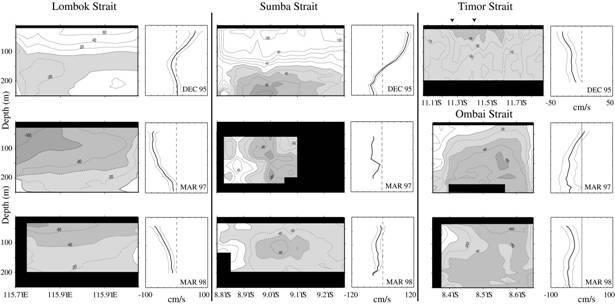
Figure 4. Tidally-corrected along-strait velocity and
section-mean velocity profiles in Lombok Strait, Sumba Strait, Ombai Strait, and
Timor Passage during December 1995 (top), March 1997 (middle), and March 1998
(lower panel), after Hautala et al. (2001). In the velocity sections, the contour
interval is 20 cm/s, and negative values are towards the Indian
Ocean and shaded. Error
bars in the velocity profiles are light lines.
Arrows show the position of the moorings deployed in Timor Passage in
1992-93 (Molcard et al., 1996).
The Timor Passage is a long, narrow trench that lies between
the south-east oriented coast of Timor and the northern
edge of the wide, shallow Northwest Australian coastal shelf (Fig. 1). The eastern-most
sill connecting the Timor Passage with the Banda Sea is
1250 m, shallower than the 1890 m western sill connecting the trench with the
southeast Indian Ocean near Roti Island.
Deployment of two year-long moorings across the western sill have been
undertaken on two occasions by the French: a total westward transport of 4.5+/-
1.5 Sv from 120 -1050 m in August 1989 - September
1990 (Molcard et al., 1994); and 4.3 +/- 1 Sv westward from 0-1250 m in March 1992-April 1993 (Molcard et al., 1996). Based on an ADCP repeat survey of
Timor Passage in December 1995 (Fig. 4), Hautala et
al., (2001) suggest that the high-velocity surface core of the Throughflow may extend further south than the Molcard et al. (1996) extrapolation allowed for, that would
lead to a much higher total transport through Timor Passage. Longer time series
from four moorings near the western sill in Timor Passage, contributed by Australia
and France as
part of INSTANT, will more fully resolve the Timor Passage contribution to the Throughflow.
2.2 To
resolve the annual, seasonal and intraseasonal
characteristics of the ITF transport and property flux.
The recent measurements around the Indonesian archipelago
revealed an unanticipated richness in the time-scales of Throughflow
variability, from intraseasonal (40-60 days) to interannual (El Niρo). The
different time scales are evident in all the transport and property fluxes and
are likely the result of remote forcing by both the Pacific and Indian
Ocean winds, and local forcing within the regional Indonesian
seas. Earlier estimates of the mean Throughflow were
wide ranging (2-22 Sv; Godfrey, 1996) in part because
of the lack of direct estimates, but also because of the real variation that
can severely alias estimates of the mean if survey periods are not long enough.
In fact, the recent estimates from the time series measurements suggest a Makassar Strait inflow transport of 9.3 Sv that is comparable to the transport sum of 10.5 Sv through the passages of the Lesser Sunda
Island chain (Fig. 1). However, these mean ITF estimates should be interpreted
with some caution as the time series were made at different times and at
different periods of the ENSO phase: the Makassar
moorings were in 1997-98; the Ombai mooring in 1996;
Timor Passage in 1989-90 and 1992-93; and Lombok
Strait in 1985.
Annual changes in the ITF arise from the
directionally-varying winds associated with the regional monsoon system. During
the northwest monsoon from December through March, surface waters flow into the
Banda Sea (Wyrtki, 1987), with
Ekman downwelling reaching
a maximum in February (Gordon and Susanto, 2001). The
flow from the Banda Sea towards the Indian
Ocean is strongest from July to September, when flow is enhanced
by the local Ekman response to the more intense
southeast monsoon (Wyrtki, 1987). Observations from
the 3-year pressure gauge data in the Lesser Sunda
outflow passages (Fig. 3; Chong et al., 2000; Hautala et al., 2001), as well as year-long current meter
data in Lombok Strait (Murray and Arief,
1988), Ombai Strait (Molcard
et al., 2001) and Timor Passage (Molcard et al.,
1994; 1996) all find maximum Throughflow occurs
during the southeast monsoon period.
Remote wind forcing from the Indian Ocean
is responsible for changes in the ITF via the generation of coastal Kelvin
waves. Anomalous wind bursts in the equatorial Indian Ocean
force an equatorial Kelvin wave that, upon impinging the west coast of Sumatra,
results in poleward propagating coastal Kelvin waves.
Westerly wind bursts force downwelling Kelvin waves
semi-annually during the monsoon transitions, nominally in May and November,
and intraseasonally in response to the Madden-Julian
Oscillation. The poleward propagation of the downwelling coastal Kelvin wave raises the sea level along
the Indonesian wave guide of Sumatra and the Lesser Sunda
Islands (Clarke and Liu, 1993; 1994), causes maximum eastward flow of the
semi-annually reversing South Java Current (Quadfasel
and Cresswell, 1992), and may temporarily reverse the
along-strait pressure gradient within the Lesser Sunda
passages. In May 1997, a semi-annually forced Kelvin wave resulted in eastward
flow of very fresh, warm water in the South Java Current (Sprintall
et al., 1999), with subsequent northward flow observed up through Lombok Strait and past the mooring in Makassar
Strait (Sprintall et al., 2000).
Remote winds in the Pacific Ocean
drive large-scale circulation that impacts the ITF on low-frequency, interannual time scales. The weakening of Pacific tradewinds that occurs during El Niρo, reduces the pressure
gradient between the Pacific and the Indian Ocean
thought to be the driving force for the Throughflow
on long time scales (Wyrtki, 1987). The changes are
of the sense that transport is smaller (larger), and the thermocline
is shallower (deeper) during El Niρo (La Niρa) time periods (Kindle et al., 1989; Meyers, 1996; Bray
et al., 1996; Fieux et al., 1996; Gordon and Fine,
1996; Potemra et al., 1997). The high resolution POP
model yields a 12 Sv annual average during La Niρa and 4 Sv average during El Niρo (Gordon and McClean, 1999).
The Arlindo mooring observations within Makassar Strait, which span the entire cycle of the strong
1997/1998 El Niρo, find a correlation (r = 0.73)
between Makassar transport and ENSO (Gordon et al.,
1999; though the time series is far too short to say this with assurance).
During the El Niρo months of December 1997 to
February 1998, the transport average is 5.1 Sv with a
corresponding internal energy heat transport of 0.39 pW,
while during the La Niρa months of December 1996 to
February 1997, the average is 12.5 Sv, a 2.5-fold
difference, with a 0.63 pW heat transport. In the
Lesser Sunda exit passages, the transport variations
estimated from the shallow pressure gauges suggest diminished flow through Lombok and Ombai Straits
during the ENSO years 1997-98, with increased flow through Timor Passage (Fig.
3, Hautala et al., 2001). This is consistent with a
20-year run of the POCM model, in which there is stronger flow through Timor
Passage and weaker flow through Ombai
Strait during ENSO events (Potemra et al., 2002), although the magnitude of the
transport changes through the two model straits do not fully compensate each
other. This change in the ratio of transport carried through each of the
Indonesian passages has been hinted at by the recent measurements, although
again, the lack of simultaneous direct measurements in the passages makes
interpretation difficult. For example, water mass analysis suggests that during
La Niρa time periods, when Makassar
Strait Throughflow is maximum, it appears that
additional, albeit small, amounts of North Pacific thermocline
water may enter the Banda Sea via the Lifamatola
Passage from the Maluku Sea (Gordon and Fine, 1996).
In order to resolve the time scales of variability in the
ITF transport and property flux, it is necessary for simultaneous direct
measurement within the major Indonesian Throughflow
passages, for a minimum period of three years. The characteristics of the
annual, semi-annual and intraseasonal flow during the
deployment period will be directly captured. While a 3-year time series alone
is not sufficient to comprehensively resolve the interannual
signal, combination with the earlier direct measurements in Makassar
Strait (Gordon et al., 1999), Lifamatola Passage (van
Aken et al., 1988), Lombok
Strait (Murray and Arief, 1988), Ombai
Strait (Molcard et al., 2001), and Timor Passage (Molcard et al., 1994, 1996), can help put the observational
period into perspective. Further, with simultaneous monitoring the propagation
of features such as coastal Kelvin waves along the wave guide of the Lesser Sundas and up into Makassar
Strait and the internal seas should be readily apparent. Finally,
the transport partitioning through the various passages can be explicitly determined.
2.3 To
investigate the storage and modification of the ITF waters within the internal
Indonesian seas, from their Pacific source characteristics to the Indonesian Throughflow water exported into the Indian Ocean.
The different monitoring programs within the Indonesian
region indicate large differences in peak transport timing between the inflow
and outflow straits. For example, while Gordon et al. (1999) found maximum
transport through Makassar Strait during the
northwest monsoon of 1996-1997, flow observed during the SPGA leaving the
interior seas for the Indian Ocean was minimum during the same period (Hautala et al., 2001). The phasing differences are most
likely due to storage in the Banda Sea. Assuming a
two-layer ocean, Gordon and Susanto (2001) find Banda
Sea surface water divergence, estimated from satellite altimetric
data, correlates reasonably well with a 3 month lag of the export of upper
ocean water through the Lesser Sunda Island exit
passages, as estimated by the SPGA. It appears that the Banda Sea
acts as a reservoir, filling up and deepening the thermocline
during the northwest monsoon. During the more intense southeast monsoon, Ekman flow in the Banda Sea combined
with the lower sea level off the south coast of the Lesser Sunda Islands, are more conducive to drawing
waters into the Indian Ocean (Wyrtki,
1987). The storage of mass and heat within the internal Indonesian seas will
dramatically affect the interpretation of the measurements of the Throughflow if made over short time scales.
The Banda Sea is also the primary site
for conversion of the ITF profile from Pacific into the distinct Indonesian
Sea stratification, which is then
exported into the Indian Ocean (Ffield
and Gordon, 1992). A series of CTD stations from the Arlindo
1993-94 cruises shows the attenuation and modification of the temperature and
salinity profiles from the North Pacific salinity-maximum (100-150 m) and
salinity-minimum core layers (250-350 m) with distance from the Makassar Strait (Ilahude
and Gordon, 1996). The temperature and salinity stratification as well as the
SST, are significantly altered by the strong air-sea fluxes, seasonal
wind-induced upwelling and large tidal forces within the Banda Sea
(Ffield and Gordon, 1992; 1996; Hautala
et al., 2001). Besides the vertical mixing, the attenuation of the salinity
minimum is also due to the isopycnal injection of the
more saline water from the South Pacific that enters the Banda Sea
through Lifamatola Passage (Gordon and Fine, 1996; Hautala et al., 1996).
Hence it appears that both the composition and magnitude of
the stored waters within the Banda Sea could have a
significant impact on the Indian Ocean heat, freshwater
and mass budgets. For example, Hautala et al. (2001)
suggest that an imposed transport imbalance of 5 Sv,
consistent with that determined by Gordon and Susanto
(2001) on seasonal time scales, affects the temperature at thermocline
depths by about 5°C. Convergences and divergences of this magnitude can have a
substantial impact on thermocline stratification, and
thus may affect SST by changing the temperature of the water being entrained
into the mixed layer. Because of the
possibility of different sampling biases in the previous measurements of the
inflow and outflow straits, and the relatively short time series, we cannot as
yet unambiguously determine transport imbalances into and out of the internal
seas. Part of the apparent differences in transport timing between the inflow
and outflow straits may be due to the nature of the measurements: the Makassar instruments were largely below 200 m,
whereas the SPGA in the outflow straits samples the upper Throughflow.
Another cause for the difference in peak-to-peak timing between transport
through Makassar Strait and
the exit passages, may be the contribution to the ITF
through the seas and passages directly north of the Banda Sea,
the so-called eastern route for the ITF. The significant phase differences
between the inflow and outflow straits need to be tested with simultaneous
direct measurements of both surface and subsurface velocity and property
measurements in all the major Throughflow passages,
over at least a 2-3 year time period, as proposed here in the INSTANT program.
Combined with remotely-sensed SST, altimeter and scatterometer
data, this should enable us to address the issues of implied local storage
within the Indonesian seas, along with the changes in the water mass
characteristics in the internal basins that may occur through mixing.
2.4 Contribute to the design of a cost-effective, long-term monitoring
strategy for the ITF
Techniques for developing proxy-ITF monitoring are
ultimately needed in order to develop cost-effective, long-term ITF transport
information. All measurements within the Indonesian passages require
assumptions about the cross-passage vertical structure to link their data to
the full passage velocity and transport information required to determine the
ITF transport of mass and heat. This is true whether the observations are
direct current meter measurements from single mooring deployments, or indirect
measurements that need geostrophic assumptions. In
addition, many observational programs can be logistically difficult and
expensive to maintain over time-scales long enough to be important to climate
variability. The challenge is to develop a relatively inexpensive but effective
network for monitoring the ITF over long time-scales, that
adequately captures the dominant time scales of throughflow
dynamics, and in turn, improves our ability to understand regional climate variability.
Given the large amount of subsurface temperature data
collected in the region along the XBT transects, as well as from the discrete
moorings in Makassar Strait,
one feasible approach for an ITF proxy-indicator comes from linking changes in
the thermal structure to ITF transport. The Arlindo Makassar Strait time series reveals that the ITF transport
is linked to thermocline depth (Fig. 2a): transport
is smaller and thermocline shallower during El Niρo (Bray et al., 1996, 1997; Meyers, 1996; Ffield et al., 2000). The correlation between variability
in the average thermocline temperature to variability
in the southward Makassar
transport is r=0.67 (Ffield et al., 2000). Using
nearly 15 years of XBT data, Ffield et al. (2000;
Fig. 2b) show that the Makassar
upper thermocline temperature is highly correlated
with ENSO: 0.77 for the Southern Oscillation Index; -0.80 for NINO3 SST
anomaly, and -0.82 for NINO4 SST anomaly. The correlations increase when the
ENSO time series are lagged a month or so. Thus the Makassar temperature field - when coupled with
the throughflow - transmits equatorial Pacific El Niρo and La Niρa temperature
fluctuations into the Indian Ocean.
Another feasible technique for proxy ITF monitoring comes
from linking the changes in ITF transport to the cross-strait changes in
observed or remotely sensed sea level, or (equivalently) pressure gauge data.
The technique has been successfully employed from observed shallow pressure
variations in the SPGA, deployed in the exit passages of Indonesia from 1996-99
(Fig. 3; Chong et al., 2000; Hautala
et al., 2001; Potemra et al., 2002). The transport
fluctuations through the straits are calculated assuming geostrophy
(confirmed by Potemra et al., 2002) to relate the
cross-strait pressure gradient to velocity (Hautala
et al., 2001). Using sea level to estimate the transport has two real
advantages: the geostrophic estimate is an integral
across each passage and the measurement is simple and relatively inexpensive to
make. The disadvantage is that the vertical position of the gauges relative to
the geoid is unknown, so only sea level fluctuations
are determined. However, Hautala et al. (2001) used
the information from concurrent repeat ship-board ADCP surveys of 1-2 days
duration across the same passages as the pressure sensors (Fig. 4) to calibrate
the sea level fluctuations, by scaling the inferred surface flow to the
tidally-corrected ADCP measured surface flow. This yields the absolute shallow
velocity and transport estimates shown in Fig. 3. For the INSTANT program, we propose to
redeploy the SPGA simultaneously with the mooring deployments in Lombok Strait,
Ombai Strait
and Timor Passage. Instead of using the repeat ship-board ADCP surveys to
calibrate the sealevel fluctuations, in INSTANT we
will use the 3-year time series from the concurrent mooring data. This approach
has numerous advantages: the tidal signal, which is the largest source of error
(10-20 cm/s) for the absolute velocity measurements (Hautala
et al., 2001), can be more accurately resolved; the mean estimate and
variability of the throughflow is available over at
least an annual time-scale; the mooring data give valuable information about
the variability of the cross-passage vertical structure over longer
time-periods and the deeper flow below the upper 250 m and so not measured by the ADCP.
Satellite altimetric measurements
of sea surface height may also provide an effective proxy approach for
determining ITF transport (see Potemra, 1999). Susanto et al. (1999) find a high correlation (r=0.7)
between western Pacific altimetric sea surface height
and Makassar Strait transport.
Susanto et al. (1999) found their mode 1 (68% of the
total variance), from a complex singular value decomposition of the altimetric sea surface height within the internal
Indonesian seas, is well correlated with the NINO3 index. Mode 2 (15% of the
total variance) is associated with the annual (monsoon) frequency. Similarly, Waworuntu (1999) shows a strong signal in the altimetric sea surface height signal of the central Banda
Sea during the 1997-98 ENSO event, that corresponds to the same trends in Throughflow transport observed in Makassar
Strait by Gordon et al. (1999): low (high) sea level corresponding to maximum
(minimum) transport. Recently Gordon and Susanto
(2001) determined that satellite altimetry may serve as an effective means for
monitoring the Banda Sea surface layer divergences that
may be a good indicator of storage in the internal seas. Finally, continuous,
high-quality tide gauge data along the islands in the internal seas as provided
by the Bakosurtanal (see Table 1 for supporting
measurements) would be particularly useful for determining the dominant tidal
frequencies needed for ground truthing the satellite
data. The direct measurements of the ITF by current meter and ADCP moorings,
such as proposed for INSTANT, will be necessary to help mold and ground-truth
the required algorithm needed to convert the proxy data to ITF transport
information. A two-three year coherent process study throughout the Indonesian
region is needed to clearly identify the ITF proxies necessary to provide
sustained observations.
3. INSTANT Mooring Array (2003-2006)
Attempts to measure the ITF have been piecemeal: individual
channels measured at different times. Thus we have no unambiguous picture of
the mean and variable nature of the ITF. The proposed INSTANT program has the
central goal of alleviating this deficiency, and to establish proxy indicators
of the ITF which may be suitable for long term monitoring. Here we describe the
design and instrumentation of the Makassar,
Lombok and Ombai
moorings as part of the U.S.
involvement in the INSTANT program (Table 1).
3.1 Makassar Strait
We propose to install two moorings at the same positions as
the Arlindo moorings of 1996-1998 (Fig. 1), with
temperature, conductivity, and pressure sensors, current meters, and up- and
down-looking ADCPs (Table 2). The 500-650 m deep Dewakang Sill in the southern Makassar Strait is a heavily fished region,
too broad with a labyrinth of channels to realistically measure the Throughflow. The extension of the Arlindo
MAK time series by INSTANT Makassar
time series will provide improved resolution of the interannual,
seasonal and intraseasonal signals within the throughflow and their relationship to the temperature and
salinity stratification. In addition,
essential information on the currents of the upper 200 m, not fully resolved by
MAK-1 and MAK-2, will be obtained by the paired ADCPs.
The upward looking ADCPs (RDI Long
Ranger 75 KHz) will
resolve the surface layer and upper thermocline. We suspect that the currents in the upper 100
meters vary seasonally. The downward
looking ADCPs (WH300) will resolve the middle and
lower thermocline currents. The paired ADCPs
are positioned on the mooring to profile what is hypothesized to be the maximum
in throughflow current while keeping the bulk of the
mooring flotation and associated drag in the lower speed regime of the lower thermocline.
Together, they will provide velocity profiles of the water column lying
approximately between 50 and 400 meters during all phases of the
tidally-induced mooring motion, minimizing ambiguities due to mooring blowover in this portion of the water column. Current meters at 200 (MAK West) and 750
meters correspond to instrument depths of MAK-1, 2. The current meter placed at
1500 m on MAK West will allow for investigation of a phenemenon
observed in the MAK-1,2 times series: the 750 m and 1500 m along channel
velocities, while averaging near zero, display out of phase events with speeds
of up to 20 cm/sec. The ADCPs and current meters will sample at 30 minute
intervals.
Table 2. INSTANT Mooring Array (US moorings shown in bold)
|
|
sill depth
(m)
|
width (km)
|
mooring position (°S; °E)
|
bottom
depth (m)
|
ADCP (+T)
|
currents
(C), temperature (T), salinity (S), pressure (p)
|
Makassar Strait
|
650
|
45
|
02°51.7';
118°27.5'
(MAK West)
|
2137
|
300 (up)
310 (dn)
|
T-p: 45, 60,
80, 100, 130, 150, 170, 205, 225, 245, 295, 332, 366, 434, 468; T-S-p: 115, 265; C-T-p: 40, 200, 400; C: 750, 1500
|
|
|
|
02°51.2';
118°37.7'
(MAK East)
|
1611
|
300 (up)
310 (dn)
|
T-p: 295; T-S-p: 115;
C-T-p: 400, 750
|
Lifamatola
|
1940
|
90
|
01°49;
126°57
|
1938
|
500 (up)
|
C-T-p: 600,800,1000
|
Passage
|
|
|
|
|
1500 (dn)
|
|
Timor
|
1890
|
80
|
11°09;
122°37
|
800
|
|
T: 200,300; C-T-S-p:
150, 250,400; C-T-p: 800
|
Passage
|
|
|
11°15;
122°42
|
1850
|
250 (up)
|
C-T-S-p: 150, 400, 700; C-T-p: 1000, 1500
|
|
|
|
11°25;
122°47
|
1500
|
250 (up)
|
T: 200,300;
C-T-S-p: 150, 400, 700;
C-T-p: 1000
|
|
|
|
11°35;
123°52
|
700
|
|
T: 200,300;
C-T-S-p: 150, 400; C-T-p:650
|
Ombai Strait
|
3250
|
30
|
8°
24; 125°08
|
1500
|
250 (up)
|
T:
200,300,500; C-T-S-p: 150, 400; C-T-p: 700, 1000
|
|
|
|
8°
33; 125°09
|
3200
|
250 (up)
|
T:
200,300,500; C-T-S-p: 150, 400; C-T-p: 700, 1000, 1500, 3000
|
Lombok
|
300
|
35
|
8°
24; 115°44
|
800
|
200 (up)
|
T:
175,250,300; C-T-S-p: 150, 450
|
Strait
|
|
|
8°
24; 115°54
|
1200
|
200 (up)
|
T:
175,250,300; C-T-S-p: 150, 450; C-T-p: 800
|
Fifteen Seabird SBE39 temperature-pressure recorders are
distributed on MAK West to resolve the temperature stratification with a 5
minute sampling rate from the near surface through the thermocline. Combined with the temperature and pressure sensors on
the current meters and the moored SBE37 Microcat CTDs (with sampling rates between 7.5 and 30 minutes) a
complete thermocline temperature field will be
resolved. Strong semi-diurnal tidal
currents will push the mooring over each tidal cycle, so all the sensors will
profile the temperature stratification four times daily (Ffield
et al. 2000). A nearly continuous, high
resolution, temperature-time section will be obtained. The twice-daily mooring blowover
due to the tides essentially converts the moored sensors into independent
temperature profiling devices. With the
dense suite of temperature measurements planned here, tidal aliasing is
minimized, and the Makassar Strait
temperature stratification will be well resolved in the thermocline.
3.2 Lesser Sunda Island
chain
The INSTANT mooring array includes 8 moorings within the
three major straits of the Lesser Sunda
Islands that control outflow into the Indian Ocean
through the Indonesian archipelago: Lombok Strait
(USA), Ombai Strait (USA) and Timor Passage (Australia,
France; Fig. 1,
Table 1, Table 2).
The location and number of moorings in Lombok and Ombai Straits,
and the proposed depth of the instruments are designed to capture the
full-depth velocity, temperature and salinity structure of the ITF outflow
following the rationale outlined in the scientific objectives above. They are
based on all the available information from the previous measurement programs
conducted within the Lesser Sunda export passages.
Thus, two moorings are proposed in both Lombok and Ombai Straits: on the eastern and northern sides,
respectively to capture the expected
reversals due to the impact of the South Java Current, and along the western
and southern sides to resolve the consistent intensification of ITF outflow
(Fig. 4; Hautala et al., 2001). In Lombok Strait,
the moorings will be located close to the northern moorings of Murray and Arief (1988). It may appear that measuring the ITF across Sumba, Dao and Savu Straits
at the western edge of the Savu
Sea, would be better than the
proposed measurement at Ombai
Strait on the eastern edge of the Savu Sea
(Fig. 1). However, for this proposal, deploying a mooring array across these
three wider channels is unrealistic and prohibitively expensive. In addition,
the results from the inferred SPGA transport time series, suggests that the
inflow from the eastern Ombai
Strait and outflow through the three
western straits should be balanced, at least on annual time scales (Hautala et al., 2001). As such, the 30 km wide Ombai Strait
offers a convenient choke-point to measure the ITF velocity and property
profiles as it directly exits from the Banda Sea.
Evidence from the recent measurements within the outflow
straits suggest that, although the velocity does vary with depth, most of the
transport seems to occur in a surface layer of only a few hundred meters thick.
To capture this surface flow, all U.S.
moorings will be outfitted with an upward-looking ADCP at 250 m in Ombai, and 200 m in Lombok.
The ADCP will be set at surface-tracking mode so that the measurements can be
corrected for the anticipated vertical motion due to tidal blow-over. The ADCPs have proven very successful in resolving the
highly-energetic surface flow through both Ombai Strait
and Timor Passage (Molcard et al., 1996; 2001). WMI acoustic current meters in the surface
layer at 150 m will provide backup for the ADCP. Discrete VMCM measurements
will be made at intermediate depths and with RCM-8s at depth (Table 2), to
adequately resolve the vertical velocity profile, and the lower thermocline contribution to ITF transport suggested by
recent water mass analysis (Fieux et al., 1996; Wijffels et al., 2001).
Vertical variation in velocity is accompanied by variation
in temperature and salinity. All current meters and the ADCPs will be equipped with thermistors
to resolve the temperature stratification. Additional temperature loggers will
resolve the upper thermocline (Table 2). In addition,
SBE-39 Microcat CTDs and SBE-04
conductivity sensors will be distributed on the current meters. Expected strong
tidal motion will be corrected for using co-located pressure sensors. Sampling
rate for the ADCP, current meters, temperature and
conductivity sensors will be 30 minutes. Thus properties are measured on the
same space and time scales as the currents, so that net property transports can
be estimated at all resolved frequencies.
3.3 Mooring
Deployment Timetable and Hull-Mounted ADCP and CTD Station Plan
The initial deployment of all the INSTANT moorings will be
in August 2003, recovery and re-deployment in February 2005, and final recovery
in July 2006. The cruises for the Makassar
and Sunda moorings will be coordinated so that
ship-time and port-calls are optimized (see budget justification). The
deployments and recoveries will be carried out from one of the Indonesian BPPT Baruna Jaya ships (Table 1). The Baruna Jaya ships are modern
research vessels, with ship-board ADCPs and
capabilities for undertaking a hydrographic CTD survey of the region. The aft
deck is considerable and has a large A-frame suitable for mooring work, indeed
the PIs have previous experience in mooring deployment and recovery aboard the Baruna Jaya IV: Sprintall in the South Java Current (see Sprintall et al., 1999) and Gordon in Makassar
Strait (see Gordon and Susanto, 1999; Gordon et al.,
1999). The vessel used for recovery will be equipped with suitable gear for
dragging for the mooring (trawl wire and winch) in the event both mooring
releases fail.
The cruises include ship days for CTD/ADCP surveys of the
channels. BPPT will carry out the CTD stations with their equipment and
personnel. Concurrent shipboard ADCP and CTD surveys will be taken around the Makassar and Sunda
moorings during each deployment/recovery cruise to provide important
information on top-to-bottom water mass structure, and for calibration
purposes. During the three mooring cruises we will steam back and forth across
the entire channel width operating the Baruna Jaya hull ADCP over 1 to 3 diurnal tidal cycles. In
addition, BPPT will obtain CTD stations to assess the along channel flow and
stratification as a function of distance from the channel side walls. Such data may be used as a basis for a
correction factor for the transport calculations. This will extend the
stratification data to further build the 1994-96 Arlindo
CTD time series of Makassar Strait
and the Indonesian internal seas, and of the French JADE hydrographic and the
SPGA data in the Lesser Sunda export channels.
LDEO and SIO personnel will carry out the mooring
preparation, deployments, and recoveries, with additional support via a
subcontract to the CSIRO Marine Research (CMR) mooring group, supervised by Dr.
Susan Wijffels. The mooring designs (see Budget
Justification for details) are a balance of maximizing data return, while
minimizing blow-over, costs, and complexity of deployment in consideration to
the deck space and capability of the Indonesian research vessel to be used in
the program. The CMR subcontract will provide all the mooring hardware, the
winches necessary to deploy the moorings, the bulk of the floatation, as well
as the lease of most of the instrumentation. The purchase of additional current
meters with temperature and conductivity sensors, ARGOS
beacons and acoustic releases are necessary to complete the mooring array. The
CMR subcontract for the mooring hardware and assembly makes the proposed work
not only cost-effective as few additional instruments need to be purchased, but
the CMR group brings their own experience to the project. The subcontract
includes participation by 2 CMR mooring specialists, to assist with the
cruises, who have previously worked on the Baruna Jaya IV during the Australian-Indonesian ASEAN mooring
program (see Luick and Cresswell,
2001; Cresswell and Luick,
2001). In addition, Wijffels as the CMR supervisor, will bring her own valuable scientific expertise
to the project, in the collaborative analysis of the acquired mooring data at
no extra cost to this proposal. Wijffels has a
distinguished scientific record of working in the Indo-Australian region on Throughflow problems (see attached resume): she is the
chief PI responsible for many Australian projects in the region (see Table 1),
such as the Indonesian seas XBT program, and the ARGO profiling float array in
the Indian Ocean, of which she is the Australian representative on the
International ARGO Scientific Steering Committee.
3.4 Proxy
and Supporting Measurements in the Indonesian Region
An important practical outcome of the ITF program will be to
recommend an efficient set of ongoing observations that adequately and
cost-effectively monitor the mass and property fluxes between the tropical Pacific
and Indian Oceans.
As part of this proposal, the regional Indonesian seas XBT, tide gauge, and
satellite altimetric data will be explored for their
potential as proxies of Throughflow transport (Table
1). These data have the advantage of including both short and long period
variations of the Throughflow properties, as well as
being part of ongoing monitoring programs.
If the Makassar thermocline temperature to transport to ENSO correlation
found during the Arlindo moorings can be confirmed
over longer time periods with additional mooring data, then the proxy data can
be used to estimate the Makassar
thermocline temperature, volume transport, and
internal energy transport from the mid-1980s to present.
We are also proposing to redeploy the shallow pressure gauge
array (SPGA) in the three outflow straits to thoroughly test its viability for
inexpensive and sustained monitoring of ITF variability. (The pressure gauges,
which rely on geostrophic assumptions for determining
flow, are probably inappropriate for monitoring Makassar
Strait given its proximity to the equator.) The pressure gauges
represent the least expensive component of the proposed fieldwork (< $40K).
Pressure is measured by a Paroscientific quartz
sensor, and their high precision (0.3 mbar) provides significantly increased
resolution of sea level over traditional stilling-well tide gauges. The largest
error comes from instrument drift, which at 0.3 mbar per year is much smaller
than the dynamic signal of ~10 mbar found in the outflow straits (Hautala et al., 2001). The instruments are internally recording, and easily deployed by divers as they are held in
the central column of a single-wheel anchor in a few meters water depth. In
fact five of the six anchors are already in place in the three outflow straits
from the 1996-99 SPGA deployment. No surface marker is used to locate the
anchor, in order to reduce the problem of vandalism. Rather, in the initial
deployment, an acoustic pinger will be attached to
the anchor to make finding them in later cruises less of a challenge.
4.
Data Sharing and Outreach
Data Sharing: The INSTANT data will be shared among the
participants as rapidly as preliminary numbers can be produced (anticipated
within 3 months of mooring recovery cruises). A web site will be maintained at
LDEO on which to post preliminary data. While most of the group interaction
will be achieved through e-mail, workshops and presentations to the community
are planned in conjunction with the American Geophysics Union and European
Geophysical Society meetings. In December 2002, a planning meeting will be held
during the AGU Fall meeting. In February 2006, a workshop is planned at the AGU
Ocean Sciences meeting to discuss preliminary results from the first 18 month
deployment. In April 2007, the INSTANT full 3 year results will be presented to
the community at the European Geophysical Society meeting; a special session on
the Indonesian Throughflow will be requested. Immediately prior to the EGS
meeting a workshop of all INSTANT investigators will be held in Nice. The full
INSTANT data set will be made available to the community 2 years after the
moorings final recovery.
Outreach: It is important that we convey to the public the
excitement and importance of ocean sciences in the remote, exotic reaches of Indonesia.
We plan the following activities. Susanto will give
ITF lectures to Indonesian schools and governmental agencies while he is in Indonesia
participating in the INSTANT cruises. Gordon will incorporate ITF information
in his Columbia University
undergraduate course on the Earth's Climate, offering independent research
projects. Sprintall will offer research opportunities to an undergraduate
student as part of the Scripps undergraduate research fellowship (SURF)
program. ITF exhibits are planned for Lamont (Ffield)
and Scripps (Sprintall) open houses.
5. In Conclusion:
INSTANT is an ambitious collaboration of 5 nations
(Australia, France, Indonesia, Netherlands and the USA), which is designed to
provide a time series of Indonesian throughflow transport and property fluxes,
and their variability from intraseasonal to annual
time scales, from the intake of Pacific water at Makassar Strait and Lifamatola Passage, to the Lesser Sunda
exit channels into the Indian Ocean. The collective merit for an
internationally coherent program has become strongly apparent given the
complexity and scope of the disparate nature of the present observations, and
the importance of arriving at a meaningful ITF. The international group of PIs bring their own valuable scientific expertise
to the project, and all have established good scientific track records of
working in the Indonesian region on Throughflow problems, both individually and
collaboratively as a team.
Working in remote areas of the world is always a challenge.
Recent progress in establishing official joint programs between Indonesia
and the U.S.
should be noted: both SIO and LDEO have Memorandums of Understanding with the
Agency for Assessment and Application of Technology (BPPT), the primary agency
responsible for management of the research vessels and oceanographic
development within Indonesia.
This progress, plus the PIs previous experience in conducting
oceanographic research within Indonesian waters, augers well for continuing
cooperation to do the proposed work. As the correspondence in Appendix I
indicates, Dr. Indroyono Soesilo,
the Chairman of the Marine Research and Fisheries Agency (within the newly
organized Indonesian government Department of Maritime Affairs and Fisheries)
is enthusiastic about the possibility of the proposed work. The involvement of
Indonesian scientists will benefit both the proposed program implementation and
the development of oceanographic research in Indonesia.